Antioxidant and Proliferative Activities of Bupleuri Radix Extract Against Serum Deprivation in SH-SY5Y Cells
Article information
Abstract
Objective
Bupleuri Radix (BR) is a major component of several Oriental herbal medicines used to treat stress and mental illness. There are evidences that antidepressant drugs modulate oxidative damage implicated in the pathophysiology of neuropsychiatric disorder, including depression. The aim of the present study was to investigate antioxidant and proliferative effects of BR against oxidative stress induced by serum deprivation in SH-SY5Y cells.
Methods
We examined the antioxidant effects of BR on a number of measures, including cell viability, formation of reactive oxygen species (ROS), superoxide dismutase (SOD) activity and levels of both Bcl-2 and Bax. We also investigated the effects of BR on cell proliferation using the bromodeoxyuridine (BrdU) assay, and used Western blot analysis to measure changes in expression of the cell cycle phase regulators.
Results
1) Serum deprivation significantly induced the loss of cell viability, the formation of ROS, the reduction of SOD activity, down-regulation of Bcl-2 expression and up-regulation of Bax expression. However, BR extract reversed these effects in dose-dependent manner. 2) Serum deprivation significantly reduced cell proliferation. Western blot analysis revealed that serum deprivation significantly decreased cyclinD1 and phosphorylated retinoblastoma (pRb) expression, and increased p27 expression. On the other hand, BR dose dependently reversed these effects.
Conclusion
This study suggests that aqueous extract of BR may exert potent antioxidant effects and also play an important role in regulating cell cycle progression during neurogenesis. These effects of BR may be a potentially important mechanism of antidepressant underlying the observed antioxidant and proliferative effects.
INTRODUCTION
The pathophysiology of several neuropsychiatric disorders including depression is accompanied by increased oxidative stress.1,2 Oxidative stress is caused by an imbalance between levels of free radicals production and efficiency of the antioxidant enzyme system to neutralize and eliminate reactive oxygen species (ROS). Free-radical damage by ROS, such as the superoxide anion and hydrogen peroxide, is the primary source of oxidative stress. The excessive accumulation of ROS and inefficiency of the antioxidant enzyme system can damage DNA and induce lipid peroxidation and protein modification, causing cellular dysfunction and even apoptosis.3,4 In the brain, the main antioxidant enzymes are superoxide dismutase (SOD), catalase (CAT) and glutathione peroxidase (GSH-Px). SOD is the most important enzyme for the detoxification of ROS and protects against oxidative stress and thus can help prevent neuronal cell apoptosis.5 Some antidepressant have been demonstrated to up-regulate activities of SOD, CAT, glutathione S-transferase (GST) and glutathione reductase (GR), as a possible target in the action of antidepressants, in animal model of stress and patients with depression.6-8 Moreover, in vivo study demonstrated that antidepressants can upregulate antiapoptotic protein B cell lymphoma protein-2 (Bcl-2) and downregulate proapoptotic Bcl-2-associated X protein (Bax).9 Therefore, antidepressants may exert neuroprotective effects by reducing apoptotic cell death via augmentation of antioxidant activity. This enhanced neuroprotection may contribute to the therapeutic effects produced by antidepressant drug treatment.
Herbal medicines have been used for centuries in a number of Asian countries for the treatment of various neuropsychiatric disorders. In particular, Kami-shoyo-san (KSS) is a popular traditional medicine used in Korea, Japan, and China for treating stress-related neuropsychiatric disorders such as depression or anxiety.10,11 We previously reported that KSS, a combinatorial preparation of Paeoniae Radix, Bupleuri Radix, Atractylodis Macrocephalae Rhizoma, Liriopis Tuber, Angelicae Gigantis Radix, Hoelen, Menthae Folium, Glycyrrhizae Radix and Zingiberis Rhizoma, produces antidepressant-like effects at both behavioral and molecular levels.12 Bupleuri Radix (BR), a major component of KSS, is also frequently included in herbal formulas designed to treat stress and mental illness.13 The major chemical constituents of BR have been known to saikosaponin, adonitol, α-spinasterol and other volatile oils, and aqueous extracts of this herb have been shown to have affinity for the 5-HT1A and dopamine D2 receptor.14,15 Recently, we provided some evidences for the antidepressant-like effects of BR extract in vivo and in vitro16: BR treatment significantly reduced the immobility time in the forced swimming test in rats; BR treatment significantly upregulated CREB and BDNF expression, two major targets of antidepressant action, through actions on the phosphatidylinositol 3-kinase (PI3K)-mediated Akt/Glycogen synthase Kinase-3β (GSK-3β) signaling pathways in human neuroblastoma SH-SY5Y cells.
It is well documented that long-term (two to four week) antidepressant administration enhances cell proliferation in adult rat hippocampus, consistent with the time course for the therapeutic action of antidepressant drugs. Furthermore, antidepressant treatment is reported to block or reverse the downregulation of neurogenesis caused by stress.17 However, the molecular mechanisms that regulate these processes remain unclear. Many reports suggest that the neuroproliferative effects of antidepressants may be due to actions on the cAMP/PKA, MAPK and Wnt signaling pathways.18-20 Shors et al.21 demonstrated that inhibition of neurogenesis with a chemical cell cycle inhibitor blocked hippocampal-dependent learning. The PI3K/Akt signaling pathway promotes cell cycle progression through inhibition of GSK-3β, leading to elevated expression of cyclin D1 and phosphorylated retinoblastoma (pRb), positive G1/S phase cell cycle regulators, and reduced levels of p27, a negative regulator of the G1/S phase of the cell cycle.22 Accordingly, this signaling may regulate hippocampal neurogenesis in the adult brain and be, therefore, another potential target for antidepressant drugs.
The aim of the present study was to explore possible antioxidant activity of BR on SH-SY5Y cells after serum deprivation-induced oxidative stress by assessing changes in ROS formation, SOD activity, as well as Bcl-2 and Bax levels. Next, we determined whether BR promotes cell proliferation via modulation of the G1/S phase regulators cyclin D1, pRb and p27 in SH-SY5Y cells following serum deprivation.
METHODS
Materials
Dulbecco's modified Eagle's medium (DMEM), fetal bovine serum (FBS) and antibiotic-antimycotic were purchased from GIBCO BRL (Grand Island, NY, USA). 3-[4,5-dimethylthiazol-2-yl]-2,5-diphenyltetrazolium bromide (MTT) and dichl-orofluorescin diacetate (DCFH-DA) was obtained from Sigma. Antibodies used for western blotting were obtained from the following sources: anti-Bax and anti-Bcl-2 were obtained from Santa Cruz Biotechnology (Santa Cruz, CA, USA); anti-cyclin D1, anti-phosphorylated Rb (Ser 780) and anti-p27 from Cell Signaling Technology (Cell Signaling, Beverly, MA, USA); anti-α-tubulin was purchased from Sigma; ECL anti-mouse IgG, horseradish peroxidase linked species-specific whole antibody from Amersham Pharmacia (Amersham, Little Chalfont, Buckinghamshire, UK) and anti-goat and anti-rabbit IgG-horseradish peroxide conjugates from Santa Cruz Biotechnology. The SOD enzyme assay kit was purchased from Cayman Chemical (Ann Arbor, MI, USA). Cell proliferation was measured using the 5-Bromo-2'-deoxy-uridine (BrdU) labeling and detection kit from Roche Molecular Biochemicals (Indianapolis, IN, USA). All other chemicals were purchased from commercial sources.
Plant material
Bupleuri Radix root was authenticated by Dr. Y.-K. Park, one of the authors. A voucher specimen (BR-W-0303) was deposited in the College of Oriental Medicine, Dongguk University herbarium.
Preparation of Bupleuri Radix extracts
BR was air dried and extracts were prepared by boiling sample in 200 mL water at 100℃ for 2 h. The procedure was repeated twice. The extracts were then filtered, concentrated using a vacuum evaporator, and lyophilized into powder. The total extract yield was 20% (w/w).
Cell culture
SH-SY5Y human neuroblastoma cells, obtained from the American Type Tissue Culture Collection (Rockville, MD, USA), were cultured in medium with an equal amount of Dulbecco's modified essential medium (DMEM) supplemented with 10% heat-inactivated fetal bovine serum and 1% antibiotic-antimycotic. The cells were maintained in a humidified atmosphere of 5% CO2 at 37℃. The culture medium was changed every two or three days. BR extract was dissolved in distilled water (DW) and diluted with DMEM medium to a concentration of 1,000 mg/mL. Cells were treated with various concentrations of BR extract (10, 100, and 1,000 µg/mL).
Western blotting
Cells were plated at a density of 2×106 cells per 100-mm dish. After incubation for 24 h, cells were treated with various concentrations of BR extract for 72 h. Whole cell lysates were prepared as follows: cells were washed twice with ice-cold phosphate buffered saline (PBS). Lysis buffer [20 mM Tris-HCl, 137 mM Nacl, 10% glycerol, 1% Nonidet p-40, 0.1% sodium dodecyl sulfate (SDS), 0.5% Sodium deoxycholate, 2 mM EDTA, 1 tablet complete protease inhibitor (Roche, Laval, Quebec, Canada), 20 mM NaF and 1 mM Na3VO4] was then added, followed by centrifugation (1,000×g, 10 min, 4℃). Equal amounts of protein (20 µg) from the cell extracts in each treatment condition were separated using SDS polyacrylamide gel electrophoresis and then transferred electrophoretically onto polyvinylidene fluoride (PVDF) membranes. PVDF membranes were blocked by incubation in 5% (w/v) nonfat milk in Tris buffered saline (TBS) with 0.15% Tween 20 (TBS-T) for 1 h. After incubation with a primary antibody (Bcl-2, 1 : 500; anti-Bax, 1 : 500; anti-cyclin D1, 1 : 1,000; anti-phosphorser780-Rb, 1 : 1,000; anti-p27, 1 : 1,000; anti- anti-α-tubulin, 1 : 2,000) in TBS-T at 4℃ overnight, the membranes were washed three times in TBS-T for 10 min. The membranes were then incubated for 1 h in TBS-T containing the horseradish peroxidase-conjugated secondary antibody (anti-cyclin D1, 1 : 2,000, anti-phosphor-ser780-Rb, 1 : 2,000, and anti-p27, 1 : 2,000; ECL anti-mouse IgG for anti-Bcl-2, 1 : 500, anti-Bax, 1 : 500, and anti-α-tubulin, 1 : 10,000). Immunoreactive bands were visualized and quantified using ECL+ Western blotting reagents, with chemifluorescence detected by Las-3,000 Image Reader (Fuji Film, Tokyo, Japan) software. Protein levels were normalized to the housekeeping protein α-tubulin to adjust for variability of protein loading and expressed as the percentage of vehicle control (deemed to be 100%).
Cell viability assay
Cell viability was measured using the MTT assay. SH-SY5Y cells were seeded on 96-well plates at a density 2×104 cells per well, and then cultured for 72 h in media with or without serum, with various concentrations of BR extract dissolved in DW. Cell viability was then determined using colorimetric measurement of the reduction product of MTT. The culture medium was removed, 100 µL of a DMSO solution was added to each well, and the plates incubated at 37℃ for 4 h to dissolve the formazan that had formed. Reduced MTT was measured on an ELISA reader (SLT Spectra; SLT Instruments, Sal-zburg, Austria) at a wavelength of 570 nm. Values for each treatment group are expressed as a percentage of the control.
ROS activity
To measure the ROS production in SH-SY5Y cells produced by serum withdrawal, we used the DCFH-DA method described as follows. DCFH-DA is a fluorescent dye that crosses the cell membrane and is enzymatically hydrolyzed by intracellular esterases to nonfluorescent 2',7'-dichlorofluorescein (DCFH). Cells were plated at a density of 1×104 cells per 96-well dish. After 48 h, cells were treated with DCFH-DA at a final concentration of 100 µM in DMEM for 1 h at 37℃ and then washed with PBS. The cells were then treated with various concentrations of BR extract. ROS levels were measured 72 h later using a kinetic microplate reader (Bio-teksynerge HT, Instruments, USA) at wavelengths of 485 nm (excitation) and 535 nm (emission). Values for each treatment group are expressed as a percentage of the control.
SOD enzyme activity
Cells were plated at a density of 1×106 cells per 100-mm dish. After 24 h of incubation, the cells were treated with various concentrations of BR extract for 72 h. For whole cell lysates, the cells were washed twice with ice cold phosphate buffered saline (PBS). Lysis buffer was added, followed by centrifugation (1,000×g, 10 min, 4℃). Equal 20 µL lysate aliquots were used for determination of SOD enzyme activity as per the manufacturer's instructions (Superoxide Dismutase Assay Kit, Cayman Chemical Company, USA). Reduced SOD was measured using an ELISA reader (SLT Spectra; SLT Instruments, Salzburg, Austria) at a wavelength of 450 nm. Values for each treatment group are expressed as a percentage of the control.
Cell proliferation assay
The BrdU labeling and detection kit (Cell Proliferation ELISA, Roche, Germany) was used for the quantitative determination of BrdU incorporated into cellular DNA. Cells were grown in a 96-well microplate and were incubated with BR extract at the concentrations indicated for 72 h, after which the BrdU assay was performed. The extent of the reaction was measured using an ELISA reader at 370 and 492 nm (Bio-teksynerge HT, Instruments, USA). Values for each treatment group are expressed as a percentage of the control.
Statistical analysis
Statical analyses were carried out using repeated-measures one-way ANOVA followed by Scheffe's post-hoc tests. p values ≤0.05 were deemed to be statistically significant.
RESULTS
Antioxidant effects of Bupleuri Radix
Effects of Bupleuri Radix on cell viability
To assess the protective effect of BR on serum deprivation-induced cytotoxicity, we analyzed cell viability using the MTT assay. Serum deprivation reduced cell viability to about 38% of control values (p<0.01)(Figure 1). This toxic effect of serum deprivation was significantly attenuated by BR treatment in a dose-dependent manner (p<0.01)(Figure 1).
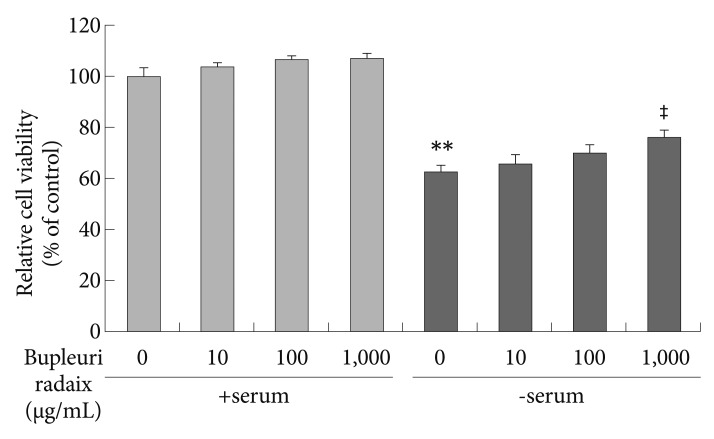
Effects of Bupleuri Radix on the cell viability in SH-SY5Y cells. Cells were treated with different doses of Bupleuri Radix for 72 h with (+serum) or without serum (-serum), after which the 3-[4,5-dimethylthiazol-2-yl]-2,5-diphenyltetrazolum assay was performed. Values are expressed as a percentage of control (serum-normal, no drug treatment) and represent means±S.E.M. from the three independent experiments. **p<0.01 vs. untreated control, ‡p<0.01 vs. serum starved-alone.
Effect of Bupleuri Radix on ROS formation and SOD activity
To examine whether the protective effect of BR on serum deprivation-induced toxicity are mediated through antioxidant actions, intracellular ROS levels were quantified using the DCFH-DA assay and SOD activity was measured using a commercially available kit. Following serum withdrawal, ROS levels were greately increased, to about 442 % of control values (p<0.01)(Figure 2A). BR treatment dose-dependently suppressed serum withdrawal-induced ROS generation (p<0.01)(Figure 2A).
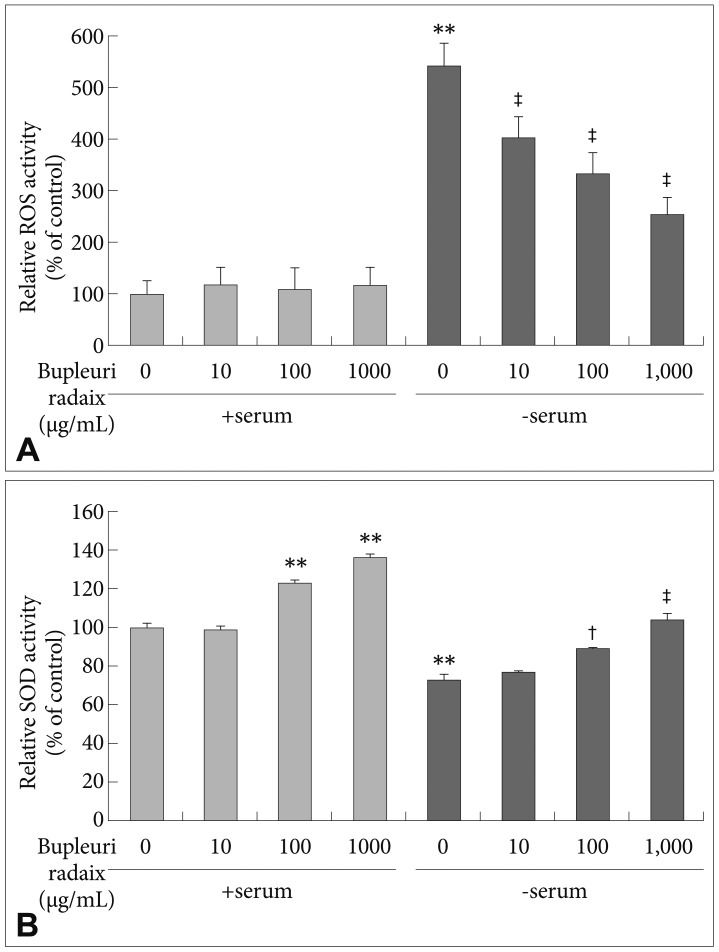
Effects of Bupleuri Radix on ROS formation and SOD activity in SH-SY5Y cells. Cells were treated with different doses of Bupleuri Radix for 72 h with (+serum) or without serum (-serum). The fluorescence intensity of dichlorofluorescin diacetate was measured (A). SOD activity was assessed as described in the Methods (B). Values are expressed as a percentage of control (serum-normal, no drug treatment) and represent means±S.E.M. from the three independent experiments. **p<0.01 vs. untreated control, †p<0.05 vs. serum starved-alone, ‡p<0.01 vs. serum starved-alone. ROS: reactive oxygen species, SOD: superoxide dismutase.
SOD activity was decreased to 28% of control levels after serum deprivation (p<0.01)(Figure 2B). BR treatment prevented the serum withdrawal-induced decrease in SOD activity in a dose-dependent manner (p<0.01)(Figure 2B). Moreover, treatment with BR at 100 µg/mL and 1,000 µg/mL significantly increased SOD activity by 23% and 36%, respectively, under serum-normal conditions (p<0.01)(Figure 2B).
Effects of Bupleuri Radix on the levels of Bcl-2 and Bax
We also investigated whether BR has antiapoptotic effects on Bcl-2 and Bax expression under oxidative stress conditions caused by serum deprivation. Bcl-2 expression was significantly reduced by about 34% in serum-deprived cells, compared to control conditions (p<0.01)(Figure 3A). BR treatment restored Bcl-2 expression almost to control values (p<0.05 or p<0.01)(Figure 3A). In the presence of serum, treatment with BR increased Bcl-2 expression in dose-dependent manner (p<0.05 or p<0.01)(Figure 3A). Conversely, serum deprivation increased Bax by 84%, compared to control values (p<0.01)(Figure 3B), and BR treatment attenuated the serum withdrawal-induced increase in Bax expression (p<0.01) (Figure 3B). BR treatment also significantly reduced Bax levels under serum-normal conditions (p<0.01)(Figure 3B).

Effects of Bupleuri Radix on Bcl-2 and Bax expression in SH-SY5Y cells. Cells were treated with different doses of Bupleuri Radix for 72 h with (+serum) or without serum (-serum). Cell lysates were analyzed by SDS-PAGE and Western blotting with anti-Bcl-2 (A) and anti-Bax (B) primary antibodies. A representative image and quantitative analysis normalized to the α-tubulin band are shown. Values are expressed as a percentage of the control (serum-normal, no drug treatment) and represent means±S.E.M. from the three independent experiments. *p<0.05 vs. untreated control, **p<0.01 vs. untreated control, †p<0.05 vs. serum starved-alone, ‡p<0.01 vs. serum starved-alone. Bcl-2: B cell lymphoma protein-2, Bax: Bcl-2-associated X protein.
Cell proliferative effect of Bupleuri Radix
Effects of Bupleuri Radix on cell proliferation
To examine the effect of BR on cell proliferation, we used the BrdU incorporation method, which detects cell division by measuring new DNA synthesis. As shown in Figure 4, under serum-free conditions BrdU incorporation significantly decreased to about 23% of control values (p<0.01). However, under the same conditions BR treatment stimulated BrdU incorporation in a dose-dependent manner (p<0.01)(Figure 4). In the presence of serum, BR at 1,000 µg/mL increased BrdU incorporation by 20% compared to control levels (p<0.01).
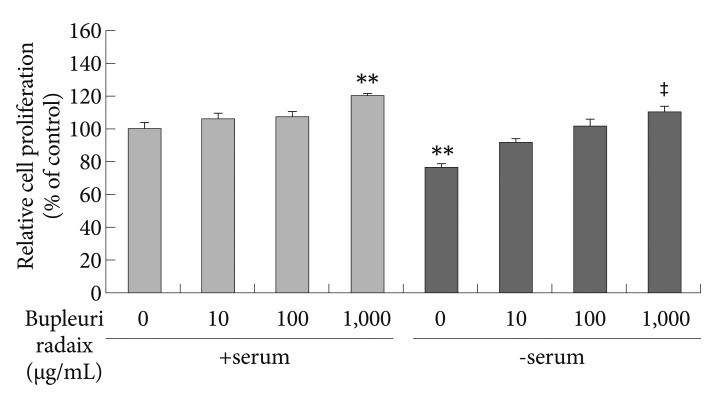
Effects of Bupleuri Radix on SH-SY5Y cell proliferation. Cell were treated with different doses of Bupleuri Radix for 72 h with (+serum), or without serum (-serum), after which the BrdU assay was performed. Values are expressed as a percentage of control (serum-normal, no drug treatment) and represent means±S.E.M. from the three independent experiments. **p<0.01 vs. untreated control, ‡p<0.01 vs. serum starved-alone. BrdU: bromodeoxyuridine.
Effects of Bupleuri Radix on cyclin D1, phosphorylated Rb, and p27 expression
As shown in Figure 5, serum deprivation reduced cyclin D1 and pRb expression to 54% and 33% of control, respectively (p<0.05 and p<0.01)(Figure 5A and B). However, under the same conditions BR treatment significantly increased cyclin D1 expression (p<0.01)(Figure 5A) and pRb expression (p<0.05)(Figure 5B), as compared to the control. In addition, BR treatment (1000 µg/mL) increased pRb levels by 19% under the serum-normal condition (p<0.05)(Figure 5B).

Effects of Bupleuri Radix on cyclin D1, phosphorylated Rb, and p27 expression in SH-SY5Y cells. Cell were treated with different doses of Bupleuri Radix for 72 h with (+serum) or without serum (-serum). Cell lysates were analyzed by SDS-PAGE and Western blotting with anti-cyclin D1 (A), anti-pRb (B), and anti-p27 (C) primary antibodies. A representative image and quantitative analysis normalized to the α-tubulin band are shown. Values are expressed as a percentage of control (serum-normal, no drug treatment) and represent means±S.E.M. from the three independent experiments. *p<0.05 vs. untreated control, **p<0.01 vs. untreated control, †p<0.05 vs. serum starved-alone, ††p<0.01 vs. serum starved-alone. pRb: phosphorylated retinoblastoma.
Seurm deprivation also upregulated the cyclin-dependent kinase inhibitor p27 (p<0.05)(Figure 5C). In contrast, stimultaneous treatment with BR prevented this increase in p27 levels, and the supressive effect was proportional to the concentration of BR used (p<0.05)(Figure 5C).
DISCUSSION
The major findings of the present study were 1) BR treatment improved cellular antioxidant defenses by affecting ROS levels, SOD activity, and Bcl-2 and Bax expression to provide protective effects against oxidative stress and oxidative stress-induced apoptosis; 2) BR treatment promoted cell proliferation via stimulation of cell cycle progression by modulating the G1/S phase regulators cyclin D1, pRb and p27.
Oxidative stress caused by serum deprivation play a causal role in the induction of apoptosis.23 Cell death induced by serum deprivation usually manifests as the hallmark features of apoptosis, including DNA laddering, activation of caspases 3 and 9, cytochrome c release, increased levels of bax, and decreased Bcl-2 levels, NF-kappa B (NF-κB) binding activity, and cellular glutathione levels.24 The present study showed that serum deprivation attenuated cell viability and induced a large increase of ROS production, reduced SOD activity, the down-regluation of antiapoptotic protein Bcl-2, and up-regulation of pro-apoptotic protein Bax in SH-SY5Y cells.
Antioxidant effects are another putative mechanism of antidepressant action. Previous studies have shown that antidepressants drugs can modulate depression-induced oxidative stress in vivo.7,25 Other reports have suggested that the neuroprotective actions of antidepressant drugs, such as amitriptyline, venlafaxine and fluoxetine, rely on upregulation of SOD activity.26,27 In addition, elevated levels of ROS can lead to apoptotis.28 Bax and Bcl-2 play key roles in the effects of ROS on the mitochondrial apoptotic pathway.29,30 Thus, whereas Bcl-2 prevents apoptosis via blockade of ROS production in mitochondria, Bax increases mitochondrial ROS production and promotes apoptosis.29,30 The present study demonstrated that BR blocked cell death, ROS production, the reduction of SOD activity, overexpression of Bax and reduction of Bcl-2 following serum withdrawal-induced oxidative stress. Consistent with these results, one study reported that the antidepressant drugs amitriptyline and tanylcypromine prevented ROS formation, glutathione depletion, Bax overexpression and Bcl-2 reduction due to MPP+ toxicity in differentiated PC12 cells.31 Based on these findings, we postulate that the effects of BR on serum withdrawal-induced oxidative stress can be partially explained through alteration of the balance between Bcl-2 and Bax, and that BR may exert neuroprotective effects via its antioxidant and antiapoptotic properties.
CREB and BDNF are known to play prominent roles in neurogenesis. As such, upregulation of CREB and BDNF could mediate the effects of antidepressant treatment by increasing neurogenesis and modulating survival signals.32 Supporting this notion, Monstany et al.20 provide evidence that antidepressant drugs enhance hippocampal cell proliferation. They reported an increase in nuclear β-catenin and cytosolic Akt levels in venlafaxine-treated animals, suggesting that antidepressants activate the intracellular signaling through Wnt (β-catenin translocation) and Akt pathways, which could then increase the expression of genes regulating the cell cycle. β-catenin, in particular, has been shown to modulate the cell cycle regulators myc and cyclin D1.33,34 Furthermore, regulation of cell cycle progression involves the PI3K/Akt/GSK-3β pathway. Thus, PI3K/Akt-mediated phosphorylation of GSK-3β leads to nuclear accumulation of cyclinD1, promoting cell cycle progression, and enhances degradation of cyclin-dependent kinase inhibitor p27, a negative regulator of the cell cycle.22,35 During the G1/S phase, Rb phosphorylation releases the E2F transcription factor from an inhibitory Rb complex, which then activates genes required for cell cycle progression.36 The present study demonstrates that BR extract were highly effective on preventing the reduction of cell proliferation and cyclin D1 and pRb expression and the elevation of p27 levels induced by oxidative stress in SH-SY5Y cell. According to Hse et al.37,38 the saponin-enriched BR fraction exerted antiproliferative activity by inducing apoptosis and blocking G1 phase cell cycle progression in the human lung cancer cell line, A549. This discrepancy may be attributable to differences in fraction vs. extract and cancer cell line vs. neuronal cell line. The underlying mechanism of BR extract in human neuroblastoma cells was unknown. Moreover, previous our study suggested that the antidepressant-like effects of BR may be mediated by upregulation of CREB and BDNF expression via the PI3K/Akt/GSK-3β signaling pathways.16 A growing body of evidence shows that impaired neurogenesis is implicated in the phathogenesis of depression.39,40 However, the mechanisms concerning the effect of oxidative stress on neurogenesis remain unknown. Based on these findings, we suggest that oxidative stress induced by serum deprivation may decrease neurogenesis via downregulation of cyclin D1 and pRb and upregulation of p27, and the enhancement of cell proliferation produced by BR treatment may reverse these effects via PI3K/Akt/GSK-3β signaling.
In conclusion, BR-mediated neuroprotection may be due to inhibition of the mechanisms that lead to oxidative stress-induced apoptosis. Moreover, BR may produce antidepressant-like effects by increasing neurogenesis via regulation of cell cycle progression. These findings will help to elucidate the pharmacological action of a variety of Herbal Medicine for the treatment of mental disorders.
Acknowledgments
This study was supported by a grant of the Korea Healthcare technology R&D Project, Ministry for Health, Welfare & Family Affairs, Republic of Korea (A090091 to S.-W. Park).