Alterations in Social Brain Network Topology at Rest in Children With Autism Spectrum Disorder
Article information
Abstract
Objective
Underconnectivity in the resting brain is not consistent in autism spectrum disorder (ASD). However, it is known that the functional connectivity of the default mode network is mainly decreased in childhood ASD. This study investigated the brain network topology as the changes in the connection strength and network efficiency in childhood ASD, including the early developmental stages.
Methods
In this study, 31 ASD children aged 2–11 years were compared with 31 age and sex-matched children showing typical development. We explored the functional connectivity based on graph filtration by assessing the single linkage distance and global and nodal efficiencies using resting-state functional magnetic resonance imaging. The relationship between functional connectivity and clinical scores was also analyzed.
Results
Underconnectivities within the posterior default mode network subregions and between the inferior parietal lobule and inferior frontal/superior temporal regions were observed in the ASD group. These areas significantly correlated with the clinical phenotypes. The global, local, and nodal network efficiencies were lower in children with ASD than in those with typical development. In the preschool-age children (2–6 years) with ASD, the anterior-posterior connectivity of the default mode network and cerebellar connectivity were reduced.
Conclusion
The observed topological reorganization, underconnectivity, and disrupted efficiency in the default mode network subregions and social function-related regions could be significant biomarkers of childhood ASD.
INTRODUCTION
Although no clear mechanism related to the abnormal brain development in autism spectrum disorder (ASD) has been identified, the understanding of the neurobiological causes of ASD is gradually increasing, and research on neuroimaging plays an important role [1-3]. Studies are being actively conducted to extract ASD-specific brain circuits [4-6], and functional brain networks using resting-state functional magnetic resonance imaging (rs-fMRI) have been widely used to explore features of ASD [6-8]. Altered functional connectivity in ASD has been highly reported through rs-fMRI studies [8,9], but the findings regarding under- and over-connectivity in the resting brain are inconsistent [8,10-12].
Evidence regarding abnormalities in the functional connectivity in ASD has gradually accumulated, and it has been suggested that changes in the major brain networks such as default mode network (DMN) are important in the pathogenesis of ASD [13,14]. Previous studies have reported that the DMN is one of the most informative brain networks for distinguishing between ASD individuals and healthy controls [15,16]. The DMN is a well-known brain network overlapping with the social brain network, which is activated during social cognition [17]. Several studies reported that dysfunction of the DMN is associated with social deficits in children and adults with ASD [18-21]. However, studies on the strength of functional connectivity in the DMN are inconsistent [21,22]. Moreover, the majority of the previous studies on the functional connectivity in ASD have targeted subjects aged ≥7 years [8,12], and there are insufficient studies on functional connectivity in young children with ASD. Since ASD is an early onset disease and brain development is fundamental for disease onset [23], it is important to explore the brain network connectivity at a younger age.
In general, functional connectivity based on correlation using resting state fMRI was measured as the pair-wise connection between regions (or nodes). The abnormal functional connectivity in children with ASD through the direct comparison of correlation matrices with children showing typical development (TD) was inconsistent across different studies. There are several reasons for this inconsistency, such as sample heterogeneity, different network definitions such as nodes and edges, and different network thresholds. Since there is no gold standard to set a threshold, studies investigating brain networks have applied various thresholds, which may lead to inconsistent results.
In this study, we adopted topological data analysis (TDA) to bypass the threshold problem. TDA investigates the underlying structure of the brain network, which has high-dimensional relations [24]. It is also known as persistent homology, and it reveals the topological invariants, of a high-dimensional network regardless of the scale, i.e., the threshold [25]. We can also quantify the invariants from the topological space. We investigated the topological features of a brain network by measuring connected components. We measured a single linkage distance (SLD) to quantify the changes in the connected components by performing graph filtration [25]. Graph filtration can bypass the arbitrariness of thresholding for network construction. The SLD for identifying the topological features of the brain network was investigated in ASD, attention deficit hyperactivity disorder [25,26], and Parkinson’s disease [27].
This study aimed to identify and compare the abnormal functional connectivity using rs-fMRI between children with ASD and those with TD including younger age group than previous studies. We used the graph filtration approach to investigate the topological features of the brain network and a graph-theoretical approach to explore the local and nodal efficiencies of the whole brain network. We hypothesized the following: 1) children with ASD show atypical functional connectivity across the whole brain network and within the DMN regions as compared to the age and sex-matched TD children and 2) atypical functional connectivity and clinical features are significantly correlated.
METHODS
Participants
This study included 31 children with ASD and 31 age- and sex-matched children with TD. All the ASD children were initially screened by board-certified psychiatrists for children and adolescents based on the diagnostic criteria of the Diagnostic and Statistical Manual, 5th edition. They were diagnosed according to the Autism Diagnostic Observation Schedule (ADOS) [28] and Korean Childhood Autism Rating Scale (K-CARS) [29,30] at the Child and Adolescent Psychiatry Clinic in Seoul National University Hospital. The exclusion criteria for the ASD group were hereditary genetic disorder, current or past history of brain trauma, organic brain disorder, seizure, or any neurological disorder, schizophrenia or any other childhood-onset psychotic disorder, major depressive disorder or bipolar disorder, Tourette’s syndrome or a chronic motor/vocal tic disorder, and obsessive-compulsive disorder. The TD group included typically developing children without any psychiatric diagnoses. The exclusion criteria of the ASD group were also applied to the TD group with the additional exclusion criterion of ASD diagnosis.
Written informed consent was obtained from all parents or guardians, and the children provided verbal assent to participate after sufficient explanation of the study prior to enrollment. All study protocols were approved by the Institutional Review Board of Seoul National University Hospital (No. 2008-116-1150).
Clinical measures
To examine intelligence, we used the Korean Educational Development Institute-Wechsler Intelligence Scale for Children-Revised [31] for school-age children with language comprehension and the Korean-Leiter International Performance Scale-Revised [32,33] for preschool- and school-age children without language comprehension. Three of the ASD children were tested for intelligence with the Korean Wechsler Intelligence Scale for Children Fourth Edition (K-WISC-IV) [34]. Social ability and social competence were assessed using the Korean translated version of the Social Responsiveness Scale (SRS) [35]. To identify autistic properties, the K-CARS-2 was used for both the ASD and TD groups.
Image acquisition and processing
Image acquisition and preprocessing
All participants underwent T1-weighted structural imaging and rs-fMRI. Scanning was performed using Siemens Trio 3 T MRI scanner (Siemens Medical Systems, Erlangen, Germany) at the Seoul National University Hospital. We analyzed the rs-fMRI data, as the purpose of this study was to explore the differences in the brain functional connectivity between the ASD and TD children.
Some of the participants needed to be sedated with oral chloral hydrate to ensure immobility during MRI. Oral chloral hydrate was administered for sedation in 29 ASD children and 15 TD children in this study, and majority of the children with TD who underwent sedation were 2–6 years old. Therefore, we additionally analyzed the functional connectivity under sedation between 15 ASD and 15 TD children of the preschool age group.
Image parameters and detailed preprocessing are described in Supplementary Material 1 (in the online-only Data Supplement) [36-41].
Graph filtration
To investigate the topological features of the brain network with varying thresholds, we applied graph filtration to find the connected components of the brain graph with filtration. The shape of the network is represented as a single linkage hierarchical clustering and transformed into an algebraic form, a single linkage matrix (SLM) [25]. The SLM was generated by calculating the SLD of cij [25]. cij is the correlation coefficient between nodes i and j, and only positive correlation was analyzed in this study. The distance between two nodes was calculated to estimate SLD as follows:
Network efficiencies
To investigate the efficiency of the brain network, we calculated global (Eglobal), local (Elocal) and nodal efficiencies (Enodal) using Brain Connectivity Toolbox (https://sites.google.com/site/bctnet/) [42]. A graph G (N, K) comprising N nodes and K edges and a binary matrix, Aij, can be represented by a 273× 273 matrix, where
Statistical analysis
In this study, the following four measures were statistically tested: SLDij, Eglobal, Elocal(i), and Enodal(i). The linear regression model evaluated differences in SLD and network measures between the two groups. We included age and sex in the model to regress out the effects as follows:
To evaluate correlations between measures and age within a group, we performed Pearson’s correlation after partialling out of the sex effect using linear regression. Next, we investigated the association between measures and clinical scores in each of the two groups by Spearman’s correlation after regressing out age and sex effects using a linear regression model. We tested the correlation between edges that showed significant group differences and 11 clinical scores: CARS, ADOS communications, ADOS social interaction, ADOS total, SRS social awareness, SRS social cognition, SRS communication, SRS social motivation, SRS restricted interests and repetitive behavior, SRS social communications index, and SRS total. We analyzed edges/nodes that showed a significant correlation with clinical scores (p<0.05). We analyzed the group differences in the SLD in preschool-age children (age, 2–6 years; ASD group, n=15 and TD group, n=15) to determine whether the underconnectivity was due to the sedation effect. All the preschool-age children in both the groups were sedated.
RESULTS
Participant characteristics
The demographic and clinical characteristics of the ASD and TD group are presented in Table 1. The mean age of the ASD and TD group was between 5 and 6 years old (5.61± 2.53, 5.65±2.48, respectively) and the proportion of male subjects was higher than that of female subjects (19 males and 12 females in each group). The CARS and the SRS were significantly higher in the ASD group because they measure the symptom severity of ASD. The Full Scale IQ was significantly lower in the ASD group.
Group differences in network topology based on graph filtration
The full Region of interest (ROI) can be found in Supplementary Table 1 (in the online-only Data Supplement). To find significant group differences in network topology based on graph filtration, we compared the SLDs between the two groups. Increased SLDs were found in the ASD group (p< 0.01, FDR corrected). A longer SLD denotes weaker functional connectivity. In ASD, the bilateral connectivities of the dorsal part of the cingulate gyri (area 23) and precuneus (area 31) were significantly decreased, which are sub-regions of the DMN. The bilateral connectivity between the dorsal part of the cingulate gyri significantly correlated with the behavioral score, i.e., the CARS (rho=0.45, p<0.05; n=29) and SRS communication (rho=0.68, FWER p<0.05; n=17) scores, in the ASD group (Figure 1 and Table 2). As compared to the TD group, the ASD group showed decreased functional connectivity of the right inferior parietal lobule (IPL) (rostroventral area 40) with the left inferior frontal gyrus (IFG) (caudal area 45) (rho=0.68, FWER p<0.05; n=17). Moreover, the functional connectivity in the ASD group significantly correlated with the sub-scores of the SRS for communication (rho=0.68, p<0.005; n=17), awareness (rho=0.52, p<0.05; n=17), and social communication index (rho=0.54, p<0.05; n=17). The left IPL was significantly connected with the right superior frontal gyrus (SFG) (medial area 9), left IFG (caudal area 45), right superior temporal gyrus (STG) (rostral area 22), and left inferior temporal gyrus (ITG) (ventrolateral area 37) (Figure 1 and Table 2). The functional distance between the left IPL (rostroventral area 40) and left IFG positively correlated with the SRS communication score (rho=0.54, p<0.05; n=17) in the ASD group.
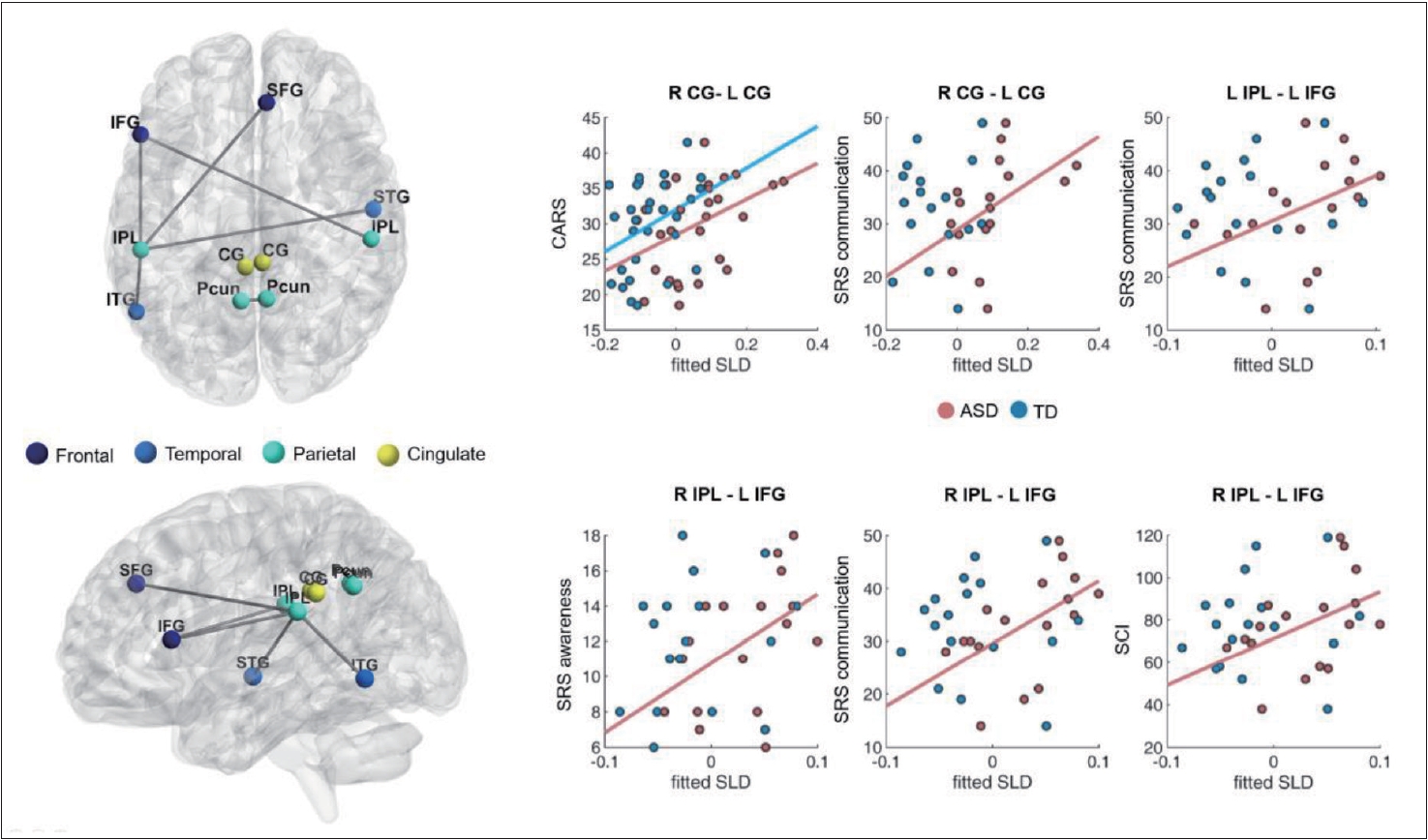
Edges with significantly decreased functional connectivity and their correlations with clinical scores. Edges with significantly increased SLD corresponding decreased connectivity are shown (p<0.01, FDR corrected) (left). The ASD children showed increased distance between the bilateral cingulate gyri and between bilateral precuneus. Edges with increased distance were also observed between the IPL and the frontal/temporal regions. Significant correlations were found between edges showing increased distance and the clinical scores (right, p<0.05). The distance between the right IPL and left IFG positively correlated with the SRS communication, SRS social awareness, and SCI. The distance between the left IPL and the left IFG positively correlated with the SRS communication. Additionally, the distance between bilateral cingulate gyri positively correlated with the CARS and SRS communication. IFG, inferior frontal gyrus; IPL, inferior parietal lobule; ITG, inferior temporal gyrus; Pcun, precuneus; CG, cingulate gyrus; SFG, superior frontal gyrus; STG, superior temporal gyrus; R, right; L, left; CARS, Childhood Autism Rating Scale; SLD, single linkage distance; ASD, autism spectrum disorder; TD, typical development; SRS, Social Responsiveness Scale; SRS awareness, Social Responsiveness Scale social awareness; SRS communication, Social Responsiveness Scale communication; FDR, false discovery rate; SCI, Social Communication Index.
Group differences in network efficiency
To identify the general network characteristics using graph theoretical measures, we examined the network efficiencies, comprising global efficiency, local efficiency, and nodal efficiency. Global efficiency significantly decreased (p<0.005) in the ASD group (Figure 2). The local efficiency of the left IFG and subgenual area 32 of the cingulate gyrus was lower in the ASD group than in the TD group (p<0.05, FDR corrected) (Table 3 and Figure 3). The local efficiency of the cingulate gyrus negatively correlated with the ADOS total (rho=-0.45, p<0.05) and ADOS social interaction (rho=-0.53, p<0.005) scores (Table 4). The ASD group showed decreased nodal efficiency in the frontal, parietal, insula, and occipital areas as compared to the TD group (p<0.05, FDR corrected) (Table 3 and Figure 3). The nodal efficiencies of the parietal and occipital areas negatively correlated with the CARS scores in the ASD group. The nodal efficiencies of the frontal and parietal areas negatively correlated and the cerebellar crus positively correlated with the ADOS sub-scores. The nodal efficiencies of the occipital and temporal areas negatively correlated with the SRS sub-scores in the ASD group (Table 4).

The global efficiency. The ASD group showed significantly decreased global efficiency as compared to the TD group (p<0.005). Violin plots of the ASD and TD groups are shown in red and blue, respectively. The individual circle denotes the global efficiency of each participant (red: ASD; blue: TD). The group average is shown as a gray horizontal line, and white circles represent the median of each group. The filled gray box in the center of each group represents the middle 50%, i.e., the range between the 25th and 75th percentiles (ASD group, children with autism spectrum disorder; Eglobal, global efficiency; TD group, children with typical development). *the significant difference was found at p<0.005.
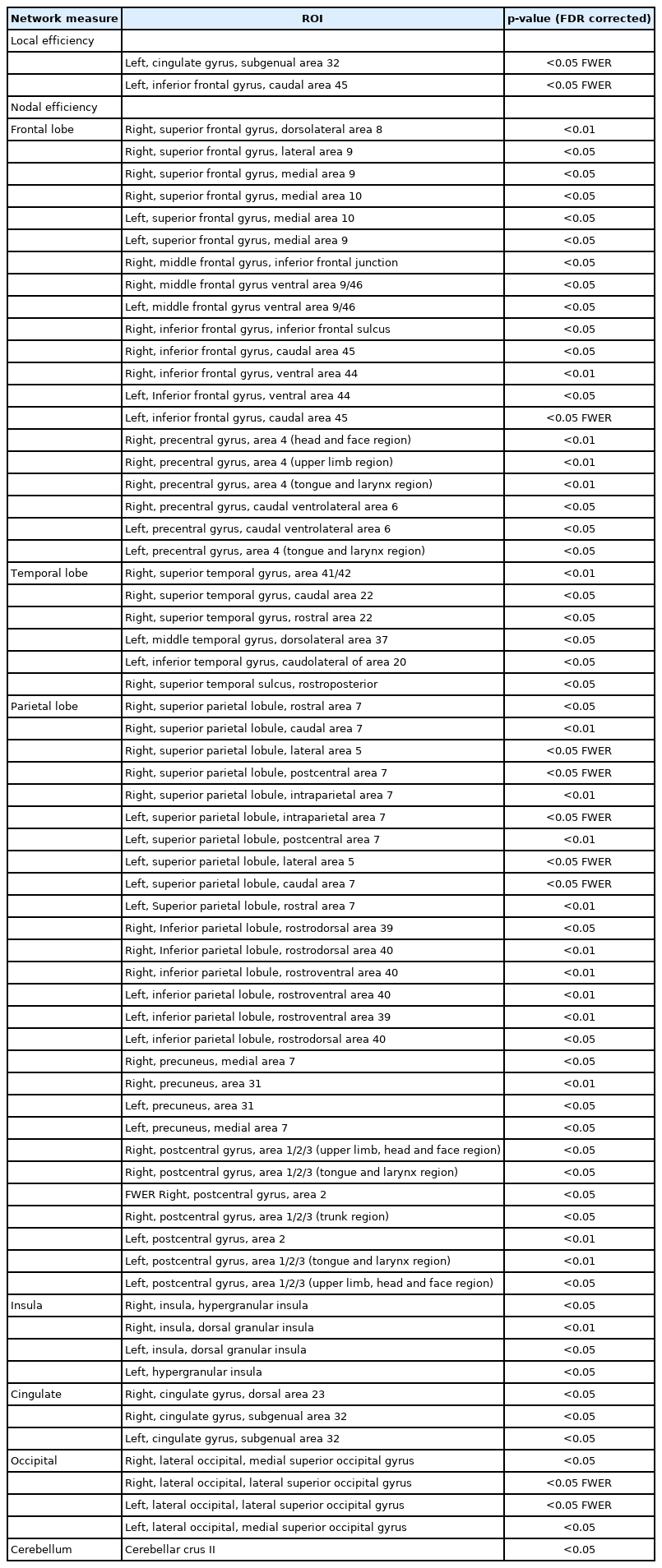
Areas showing significantly decreased local/nodal efficiency in individuals with autism spectrum disorder

Nodes showing a significant increase in the ASD group. A: The local efficiency of the left IFG and cingulate gyrus decreased in the ASD group (p<0.05, FDR corrected). B: The ASD group also showed significantly decreased nodal efficiency in the frontal, parietal, right insula, and visual areas (p<0.05, FDR corrected). IFG, inferior frontal gyrus; CG, cingulate gyrus; MFG, middle frontal gyrus; SFG, superior frontal gyrus; IPL, inferior parietal lobule; ITG, inferior temporal gyrus; CB, cerebellum; INS, insula; L, left; LOcC, lateraloccipital gyrus; MTG, middle temporal gyrus; Pcun, precuneus; PoG, postcentral gyrus; PrG, precentral gyrus; R, right; SPL, superior parietal lobule; STG, superior temporal gyrus; STS, superior temporal sulcus; ASD group, children with autism spectrum disorder; FDR, false discovery rate.
Functional connectivity and sedation
To determine whether this underconnectivity observed in our results in the ASD group was due to the sedation effect, we analyzed the group differences in the functional connectivity of the preschool children of both groups subjected to sedation during imaging. In these children (age, 2–6 years) subjected to sedation, over-connectivity was not observed. The functional connectivity between the medial (area 9) and lateral (area 10) parts of the SFG were lower in the ASD group than in the TD group (p<0.05, FDR corrected) (Supplementary Figure 1 and Supplementary Table 2 in the online-only Data Supplement). The connectivity between the right SFG (medial area 9) and right superior parietal lobule (SPL; rostral area 7) also decreased. The right SFG (medial area 10) showed decreased functional connectivity with the left SFG (lateral area 9), right STG (area 41/42 and rostral area 22), right SPL (rostral area 7), right IPL (rostrodorsal area 39), and left IPL (rostroventral area 39) (p<0.00005, uncorrected). Additionally, decreased functional connectivity between the cerebellar vermis (lobule X) and left inferior frontal sulcus was also observed. We examined the shape of the distribution of the correlation coefficients between groups with and without sedation (Supplemantary Figure 2 in the online-only Data Supplement). The kurtosis of the correlation distribution for the whole brain graph was compared between the groups with and without sedation. The peakness of the correlation distribution was higher in the sedation group than in the group without sedation (p<0.05), indicating that there were more edges with connectivity values closer to zero in the sedation group.
DISCUSSION
Decreased functional connectivity and impaired social functioning in children with autism spectrum disorder
When comparing the functional connectivity between the groups using network topology based on graph filtration, the ASD group showed decreased connectivity as compared to the TD group in the areas included in the DMN, such as the cingulate gyrus and precuneus (Table 2, Figure 1). DMN dysfunction in ASD has been consistently reported in previous studies [44-46]. In a meta-analysis published in 2019, the authors compared eight different studies that analyzed fMRI scans of target groups with various characteristics using several analytical methods of functional connectivity [11]. The results of this study showed that as compared to the TD children, the resting state functional connectivity of the DMN regions in the ASD children, such as the dorsal posterior cingulate cortex (PCC) and right medial paracentral lobule, was reduced. None of the studies included in that meta-analysis showed an increased local connectivity in ASD children as compared to TD children. In a study involving younger individuals, Yerys et al. [18] compared the resting-state functional connectivity between 22 ASD children aged 8–13 and 22 TD children matched for age, sex, and intelligence quotient. In the ASD children, the functional connectivity of the DMN core regions was reduced in the medial prefrontal cortex and PCC as compared to the TD group. In network segregation analysis, the ASD group showed a pattern of poor segregation of DMN regions as compared to the TD group. Moreover, a significant negative correlation was observed; as the functional connectivity of the DMN decreased, the clinical ASD symptoms worsened.
Similarly, this study found that as the bilateral connectivity between the cingulate gyri decreased in the ASD group, the CARS score, a clinical autism symptom score, increased (Table 2). Considering that the DMN is strongly associated with social cognition [21,47], the association between decreased connectivity of the DMN region and worsening of clinical autism symptoms in the ASD group is reasonable. In addition to the midline structures, we found decreased functional connectivity between the IPL and the frontal and temporal regions (Table 2, Figure 1). The IPL is embedded in several brain networks and converges particularly in the default mode and frontoparietal control networks [48-50]. According to previous studies, as a key node of the action observation network, IPL is known to support imitation behavior and may cause difficulties in the social communication function of ASD if impaired [51,52]. Wymbs et al. [53] reported that a decrease in the functional connectivity of the right IPL in children with ASD was associated with impairment of praxis performance and social skills when comparing the fMRI between children aged 8–12 years with ASD and with TD. Although the regions of the brain in our study did not exactly match those reported in previous studies, we found that the ASD group showed decreased connectivity as compared to the TD group in several areas linked to the IPL. We also found decreased connectivity between the left IFG and bilateral IPL in the ASD group (Table 2, Figure 1). Another study on imitation in ASD reported that IFG activity was weaker and more delayed in the Asperger syndrome group than in the TD group [54]. Furthermore, in a study of the IPL, IFG, and superior temporal sulcus (STS), i.e., brain regions commonly reported to be associated with imitation [54-56], it was found that the effective connectivity between IPL and IFG was reduced in ASD individuals. The authors suggested that the aberrant organization of imitationrelated networks may be associated with impaired development of social communication in ASD [57]. In our study, we observed decreased connectivity in the brain regions of IPL, IFG, and STG, which is consistent with the findings of previous studies. Particularly, since the decrease in connectivity between the left IFG and right IPL showed significant correlation with the communication domain of the SRS sub-scores (Table 2), the association between the corresponding brain area and social communication function can be established.
Aberrant network distribution observed in autism spectrum disorder children using graph theoretical measure
Exploring the difference in the network efficiency between the two groups by applying the graph theoretical method showed that the ASD group had significantly decreased global efficiency as compared to the TD group. Moreover, the local efficiency and nodal efficiency of the ASD group showed decreased network efficiency as compared to the TD group in areas similar to those in the SLD results shown in Table 3 and Figure 3. As the local efficiency of the left cingulate gyrus decreased, the ADOS total and ADOS social interaction scores increased (Table 4). This corroborates with the results identified in the SLD analysis; hence, the importance of the cingulate gyrus in social cognition of ASD is reinforced. The ASD children showed lower nodal efficiencies in several nodes of the frontal, temporal, and parietal areas, bilateral insula, and visual areas as compared to the TD children. On the other hand, the TD group did not show nodal efficiency lower than that of the ASD group. By applying the graph theory, it is possible to identify whether the functional networks of the brain show atypical network distribution, rather than simply distinguishing whether the functional connectivity of ASD individuals is over-or underconnected [58]. In a previous study, when the graph theoretical analysis was applied to the rs-fMRI of the ASD group and the healthy control group, analysis of global metrics showed significantly lowered clustering coefficient and characteristic path length in the ASD group, indicating that randomized organization was observed in the ASD group when compared with the control group [59]. In the same study, significantly altered local metrics were also observed (e.g., the bilateral STS, right dorsolateral prefrontal cortex, and precuneus), and the authors suggested that altered patterns of global and local metrics in ASD may underlie the social and non-social cognitive impairment [59]. We found that compared to the control group, the ASD group showed reduced nodal efficiency in brain areas related to social cognition, such as the precuneus, IPL, IFG, STG, and insula [60-62], as well as decreased nodal efficiency in the areas related to nonsocial cognitive ability in several frontal, temporal, and parietal regions of the brain [5,63-65]. Many of the results of nodal efficiency showed a significant correlation with the clinical scores of ADOS, CARS, and SRS, suggesting that the impairment of social/non-social cognitive ability affects the manifestation of the ASD symptoms, as seen in previous studies.
Decreased functional connectivity observed in preschool-age autism spectrum disorder children under sedation
Approximately half of the TD group and most of the ASD group children were subjected to sedation with chloral hydrate before imaging. Because we were concerned about medication-induced changes in the functional connectivity, we conducted an additional analysis. Moreover, when children subjected to sedation from the ASD and TD groups were analyzed separately, only preschool children aged 2–6 years were included. Thus, we not only excluded the sedation effect, but also explored the difference in the functional connectivity according to age. When comparing the preschool children under sedation between the two groups, it was observed that the functional connectivity of the DMN area remained significantly reduced in the ASD group (Supplementary Figure 1 and Supplementary Table 2 in the online-only Data Supplement). Particularly, the anterior-posterior DMN connection, such as SFG–IPL and SFG–SPL, was noticeably decreased in children with ASD. The most consistently found aberrant functional connectivity in the ASD group as compared to the TD group is the decreased frontal-posterior functional connectivity pattern. Several previous studies have shown decreased synchronization between the anterior and posterior regions [66]. In a study of anatomical and functional connectivity impairment in ASD, Just et al. [67,68] suggested that the behavioral characteristics of ASD are caused by limited communication between the frontal and posterior brain regions. They also predicted that this limitation would affect tasks that require coordination between the frontal and posterior brain regions, such as language comprehension and social interaction processes, and showed that the frontal-posterior coordination was related to these processes based on an fMRI study. Therefore, the authors hypothesized anterior and posterior underconnectivity as the underlying biological mechanism of the impaired function in ASD. Studies on the importance of anterior-posterior coordination in ASD have been conducted previously. Horwitz et al. [69] reported that the metabolic activities between several brain regions in male individuals with ASD were lower than the normal degree as observed with positron emission tomography. Particularly, they observed that the correlation between the frontal and parietal regions appeared to be reduced. In our study, the decrease in functional connectivity between the frontal and parietal regions observed in the preschool age group included the SFG, a region considered to contribute to higher cognitive function [70], and the SPL and IPL, areas known to be associated with visual motor function [71,72]. Furthermore, it has been suggested that the SPL may play an important role in motor learning and repetitive behavior in ASD [73], and the importance of the IPL area is emphasized not only in the control of various movements, but also in the impairment of the social communication function of ASD as mentioned previously [53]. Unfortunately, the correlation between the decline in the frontoparietal functional connectivity and the clinical scores in preschool-age children cannot be considered appropriate in this study due to the small sample size. As compared to the TD group, a decrease in the functional connectivity of the right SFG and right STG was also observed in the preschool-age children with ASD. The right STG is known for its important role in social cognition, which is a characteristically impaired function in ASD, and includes theory of mind, biological motion perception, and voice perception [74-76]. According to a previous study, the right STG is involved in the detailed perceptual processing of social cognition, which is associated with stronger systematization rather than empathizing, a cognitive characteristic of ASD [77].
Additionally, the functional connectivity between the cerebellar vermis and left IFG decreased in the preschool-age children of the ASD group as compared to the TD group. The cerebellum is one of the brain regions where abnormal findings are most consistently found in ASD [78], and it is extensively connected to the cerebrum; thus, cerebellar dysfunction may be implicated in several symptoms of ASD [79]. Particularly, neuropathologic observations of the vermis have been identified from the earliest days of cerebellar imaging studies of ASD [80], and vermal abnormalities are known to be associated with the emotional and behavioral deficits seen in ASD symptoms [81] Previous fMRI studies have reported abnormalities in the vermal activity of individuals with ASD [82]. For example, in a previous study, the ASD group showed a decrease in vermal medial lobule activity upon processing of irony [83], while in another study, abnormal recruitment of the posterior cerebellar vermis was observed when ASD participants processed the facial expression [84]. The underconnectivity between the cerebellar vermis and frontal region observed in this study is consistent with the findings of previous studies on ASD, suggesting that cerebro-cerebellar connectivity can be a biomarker of ASD. It is known that the integrity of the cerebro-cerebellar circuit in the early development stage is more important for cerebral development than in later development stage [85]. Furthermore, the fact that cerebellar damage in childhood can lead to poorer outcomes as compared to that in adulthood [85,86] highlights the significance of the abnormalities of cerebellar functional connectivity found in younger children with ASD. Recent studies have shown that the integrity of the cerebro-cerebellar connectivity is very crucial for early cortical development. As seen in our study, abnormalities in the cerebro-cerebellar functional connectivity found in ASD may impair the development of the cortical areas associated with motor control and language and social interaction, resulting in damage to these domains in ASD individuals [82].
Limitations
This study has several limitations. First, the sedation effect may affect the results. It is not clear how sedation-induced brain network disruptions might affect fMRI activation patterns [87], and it is possible that sedative agents may affect the fMRI results [88]. Therefore, the interpretation of the main results of this study is limited. Although we could exclude the sedation effect from the additional analysis of the preschool age group, it was insufficient because the number of participants was reduced by half. Our findings should be replicated in future studies under conditions that can control the sedation effect. Second, the sample size was relatively small. Moreover, the number of participants reduced further when only preschool-age children were considered. Future research should be conducted with a higher number of participants of the preschool-age group, as well as examination and comparison of the characteristics of functional connectivity patterns between younger and older age groups with ASD. Third, a few ASD children who took medication were included in the analysis. The drugs were anti-psychotics such as risperidone, aripiprazole and quetiapine, and a non-stimulant, atomoxetine. Although only four of the subjects took the medication, the effect of the drug on the results should have been considered. Finally, there are limitations to the results of some clinical measures of this study. The reason is not clear, but a significant number of the SRS scores were missed in the process of acquiring data from guardians. The SRS scores were measured only in 17 in the ASD group and 21 in the TD group. Therefore, there may be limitations in interpreting the correlation between the imaging results and the SRS scores. Regarding intelligence scales, the results could be inconsistent because three different scales were used depending on the age and timing of the measurement. In addition, since the ASD group in this study included relatively low functional level group, the disease itself could have an effect on low intelligence. Therefore, the results of the intelligence tests were not included in the analysis of this study.
This study included participants aged 2–11 years, who were relatively younger than those in previous studies. This study demonstrated the topological network differences using resting state fMRI between the ASD and TD groups, including the main brain networks such as the DMN and social function-related connectivity. We also obtained significant results by exploring the differences in the brain network efficiency using the graph theoretical method. These results showed significant correlations with the clinical scores of the ADOS, CARS, and SRS; thus, the abnormalities in the brain imaging results observed in ASD seem relevant. We conducted additional analyses of the ASD and TD participants of preschool age to exclude the sedation effect from the imaging results. Interestingly, decreased long-range connectivity of more brain regions and decreased cerebro-cerebellar connectivity were observed in the younger participants of the ASD group. These results confirmed that the brain functional connectivity was more impaired in the ASD children than in the TD children. Notably, our findings show that underconnectivity and disrupted efficiency in brain regions primarily related to social function, including the DMN subregions, could be significant biomarkers of childhood ASD.
Supplementary Materials
The online-only Data Supplement is available with this article at https://doi.org/10.30773/pi.2022.0174.
Region of interest
Edges showing significantly decreased functional connectivity in preschool children with autism spectrum disorder
Group differences between the preschool children (age, 2–6 years) of the autism spectrum disorder group and typical development group. The edges show significantly increased SLD in the ASD group (red: p<0.05, FDR corrected; yellow: p<0.00005, uncorrected). ASD group, children with autism spectrum disorder; FDR, false discovery rate; IFG, inferior frontal gyrus; IPL, inferior parietal lobule; ITG, inferior temporal gyrus; L, left; R, right; SFG, superior frontal gyrus; SLD, single linkage distance; STG, superior temporal gyrus; TD group, children with typical development.
Distribution of the correlation coefficients. The distribution of the correlation coefficients between the groups with and without sedation represented by light pink and light purple colors, respectively, is shown here. To compare the shape of this distribution, the kurtosis of the correlation distribution of each participant was compared between the groups with and without sedation. The mean peakness of the correlation distribution was higher in the group with sedation than in the group without sedation (p<0.05), indicating that there are more edges with connectivity values closer to zero in the sedation group (ASD group, children with autism spectrum disorder; TD group, children with typical development). *The significant difference of mean peakness was found at p<0.05.
Notes
Availability of Data and Material
The datasets generated or analyzed during the study are available from the corresponding author on reasonable request.
Conflicts of Interest
The authors have no potential conflicts of interest to disclose.
Author Contributions
Conceptualization: Narae Yoon, Hyejin Kang, Bung-Nyun Kim. Data curation: Mee Rim Oh. Formal analysis: Youngmin Huh, Hyekyoung Lee, Hyejin Kang. Funding acquisition: Dong Soo Lee, Bung-Nyun Kim. Investigation: Narae Yoon, Hyejin Kang. Methodology: Youngmin Huh, Hyekyoung Lee, Hyejin Kang. Supervision: Dong Soo Lee, Bung-Nyun Kim. Visualization: Hyejin Kang. Writing—original draft: Narae Yoon, Hyejin Kang. Writing—review & editing: Soomin Jang, Yebin D. Ahn, Chan-Mo Yang, Jung Lee, Johanna Inhyang Kim, Dong Soo Lee, Bung-Nyun Kim.
Funding Statement
This research was supported by the Bio & Medical Technology Development Program of the National Research Foundation (NRF) funded by the Korean government (MSIT) (No. 2020M3E5D9080787 to B.-N.K and 2020R1A2C2011532 to H.K.), the Technology Innovation Program (Industrial Strategic Technology Development Program) (No. 20002769, Development of Next Generation Platform for Diagnosis and Therapeutic of Attention Deficit Hyperactivity Disorder and Intellectual Disability based on Big Data) funded by the Ministry of Trade, Industry, and Energy (MOTIE, Korea) and Institute of Information & communications Technology Planning & Evaluation (IITP) grant funded by the Korea government (MSIT) (No. 2022-0-00375-001, The Development of Biomarkers based Individualized Composite Digital Therapeutics for the relief of Aberrant Behaviors in Autism Spectrum Disorder).